Terrestrial Biosphere Carbon Cycle🔗
The terrestrial biosphere carbon (TBC) cycle reflects the primary productivity of biomass, removing carbon from the atmosphere as it grows, the natural and anthropogenic carbon fluxes from biomass and soil stocks, the flux from biomass carbon to soil carbon, and the fluxes of biomass and soil carbon as methane to the methane cycle. These fluxes by land type are summed together to feed into the carbon cycle.
The Goudriaan and Ketner and IMAGE models (Goudriaan and Ketner, 1984; Rotmans, 1990) have detailed biospheres, partitioned into leaves, branches, stems, roots, litter, soil, and charcoal. To simplify the model, these categories are aggregated into stocks of biomass (leaves, branches, stems, roots) and soil (litter, soil). First-order time constants were calculated in C-ROADS assuming equilibrium in 1850 for each category land type and C-ROADS region and aggregated across regions for use in En-ROADS. Charcoal is neglected due to its long lifetime. The results are reasonably consistent with other partitionings of the biosphere and with the one-box biosphere of the Oeschger model (Oeschger, Siegenthaler et al., 1975; Bolin, 1986).
Net Primary Productivity (NPP)🔗
The natural ability of biomass to sequester carbon from the atmosphere provides a key sink in the carbon cycle. NPP is the gross primary productivity minus the autotrophic respiration. Forest, agricultural land, other land, and tundra all have primary production and respiration. Furthermore, all primary production is affected by the level of CO2 in the atmosphere (the fertilization effect). Carbon stored in biomass and soil is also released through heterotrophic aerobic and anaerobic respiration, which increases with higher temperature (increased fire, pests, decay). With the major exception of forests, all land reaches equilibrium quickly. Accordingly, the initial unit NPP of each nonforest land type is set assuming equilibrium in 1850. The flux into the biomass is equal to the flux out from aerobic and anaerobic respiration and transfer to soil is divided by the land area.
Unlike the other land types, forests have the most complex growth and the most biomass, so are treated in the most detail. Trees take up carbon through photosynthesis / primary production, and lose it through respiration, fire, being eaten by animals, decay, et cetera. Some of the carbon lost from biomass ends up in the soil through decomposition. The net of these carbon flows is that forests grow in an S-shaped pattern, slowly at first, at a high rate in middle age, and then reach an equilibrium where very high primary production is balanced by very high respiration. The growth curves, primary production, respiration and soil transfer rates are initialized and calibrated with land use harmonization (LUH), and OSCAR modeling output and compared against Houghton and Nassikas (2017) and SSP IAMs.
Initialize carbon in stocks of forest, farmland, tundra, and other biomass and soil from OSCAR 1850 output by 10 regions, disaggregated and re-aggregated to fit our 7 regions.
Initialize fractional rate of biomass and soil C respiration and transfer biomass to soil from OSCAR 1850 output.
Determine forest unit NPP Richard’s growth curve parameters for each of 7 regions.
- Set Test Pulse scenario in which all LULUCF is set to 0 EXCEPT for a pulse of 95 of mature forest in 1900; when Test Pulse = 1, all fertilization and temperature feedbacks are turned off.
- Set unit NPP inputs within ranges determined from forest analyses and assure unit NPP curves are reasonable given the types of forests in each region, e.g., more tropical in India and Other Developing A and B and more temperate in Developed.
- Iteratively adjust parameters to achieve near equilibrium prior to pulse and assure regrowth is reasonable given the types of forests in each region.
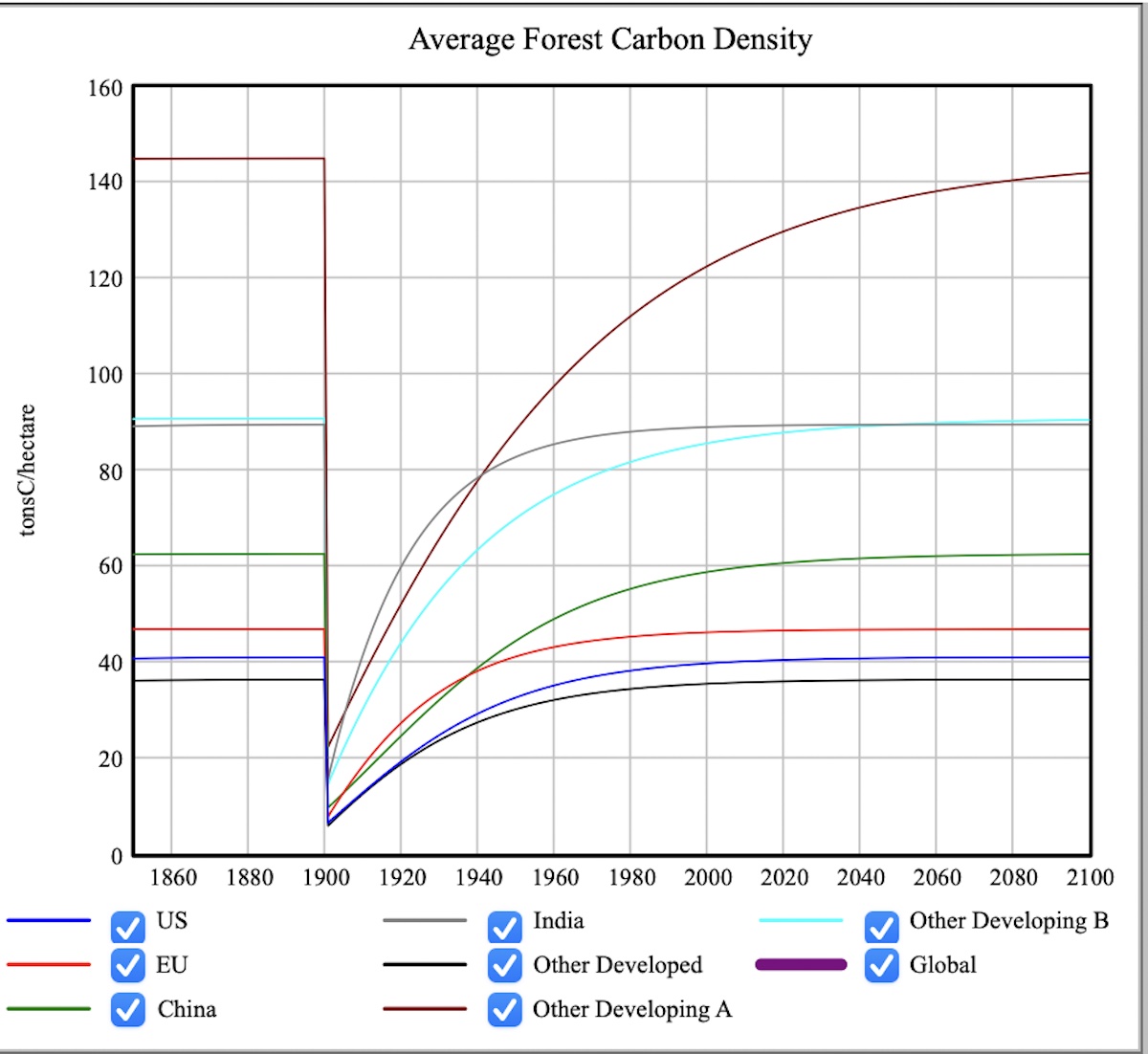
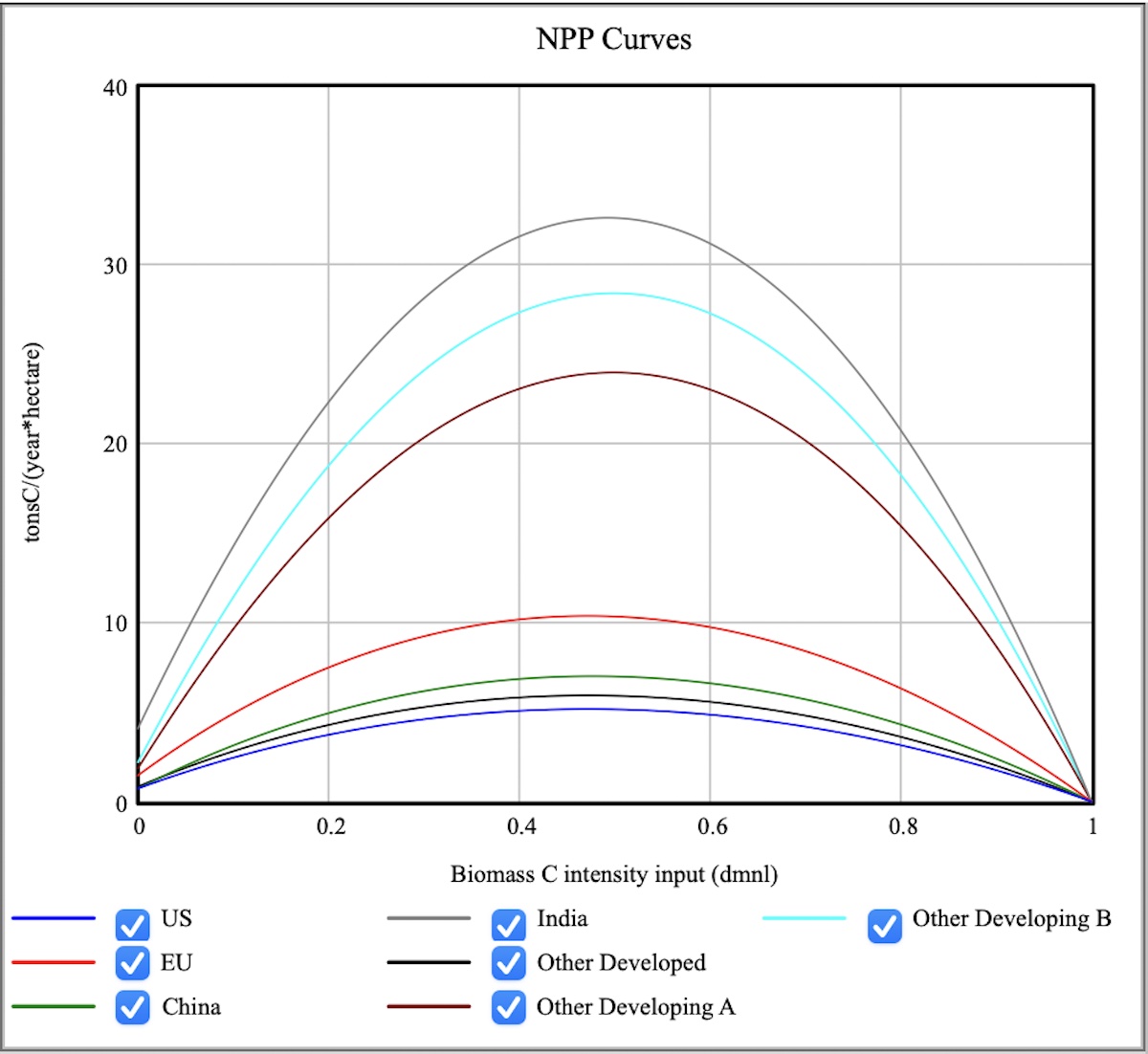
The logarithmic relationship of the uptake of C by the biosphere reflects the fact that the uptake is less than proportional to the increase in atmospheric C concentration (Wullschleger, Post et al., 1995). This formulation, though commonly used, is not robust to large deviations in the atmospheric concentration of C. As the atmospheric concentration of C approaches zero, net primary production approaches minus infinity, which is not possible given the finite positive stock of biomass. As the concentration of C becomes very high, net primary production can grow arbitrarily large, which is also not possible in reality. Accordingly, we instead use a CES production function, which exhibits the following: 1) the slope around the preindustrial operating point is controlled by the biostimulation coefficient, which can be loosely interpreted as CO2's share of plant growth (at the margin), with the balance due to other factors like water and nutrients; 2) there is a finite slope at zero CO2, such that there are no singularities; and 3) it controls saturation at high CO2.
$$ NPP = NPP_0 \biggl( 1 - \beta_b + \beta_b \frac{C_a}{C_{\mathrm{a,0}}} ^ {CO_2 \cdot sat} \biggr) ^ { \frac{1}{CO_2 \cdot sat} } $$
NPP = net primary production
NPP0 = reference net primary production
βb = biostimulation coefficient
Ca = C in atmosphere
Ca,0 = reference C in atmosphere
CO2⋅sat = coefficient that determines the rate of CO2 saturation
Natural Losses🔗
Carbon stored in biomass and soil is lost due to fire and microbial/fungal respiration. Rates of the release from each carbon stock is increased with increasing temperature change.
Carbon in both biomass and soil is also released as natural methane, entering into the methane cycle as such. The fractional rates of these releases also increase with temperature change. We assume a linear relationship, likely a good approximation over the typical range for warming by 2100. The sensitivity parameter, set by the user, governs the strength of the effect. The default sensitivity of 1 yields the average value found in Friedlingstein et al., 2006. Additionally, the rate of methane from tundra increases as temperature exceeds a threshold, representing a tipping point in the model.
Anthropogenic Carbon Fluxes🔗
Land Use, Land Use Change, and Forestry explains the land use changes and uses. Carbon emitted from LUC is a coflow of each land change, driven by the Fraction biomass C emitted and Fraction soil C emitted. The remaining carbon, i.e., 1 minus that fraction, drives the carbon transferred to the new land type.
Net removals from regrowth after harvesting and from afforestation account for the net primary productivity (NPP) and also for the carbon lost back to the atmosphere from aerobic and anaerobic respiration and to the carbon and methane cycles, respectively. In order to isolate the removals due to land changes, the model simultaneously calculates the removals for the counterfactual scenario of no land changes. Corresponding coflows, aerobic and anaerobic respiration, and transfers from biomass to soil drive the TBC cycle without harvesting and regrowth. Accordingly, the net removals due to land changes are taken as difference in net removals with and without the land changes.
The net carbon emissions from LULUCF are the gross emissions, i.e., the LULUCF released to the atmosphere from biomass and soil, minus the net removals due to the land changes.
A reduction in converting forests and in harvesting mature trees leads to a reduction in net emissions from LULUCF, eventually meaning negative emissions. Part of this is because bioenergy from wood falls - the young and medium forests cannot make up for the reduced availability of biomass from mature forests, which makes wood more expensive. Increases from the other sources of biomass (crops and waste) only partially cover the reduction from wood.
Bioenergy🔗
The amount of bioenergy used is based on the wood used for bioenergy in En-ROADS. In turn, harvesting for bioenergy removes the indicated carbon, converting any age of forest into new forest with low carbon content, or increasing the desired farmland.
LULUCF net emissions are reported in two ways, including those resulting from bioenergy and also excluding those when reporting bioenergy emissions are reported separately. Regardless of reporting, bioenergy emissions and resulting net removals are appropriately included in the TBC cycle and included as such in the main carbon cycle. Although reported as part of the energy emissions, bioenergy net emissions are not included with the Global C energy and industry emission flux of carbon into the atmosphere.
All forests supply bioenergy and wood for non-fuel products according to their carbon content. To isolate the removals due to harvesting for bioenergy, the model also calculates the counterfactual land areas and terrestrial biosphere carbon resulting from all fluxes excluding harvest and regrowth for bioenergy.